Recycling aluminum is truly marvelous from an engineering perspective: since aluminum does not change its properties when melted, it remains the same quality. Well, more or less. In theory, aluminum can be recycled infinitely without changing. Unfortunately, the reality looks different…
There is one big pitfall when it comes to using recycled aluminum for anodized products: alloying elements. In this blog post, I will share with you how different alloys affect the final anodizing results. Afterward, you know how to ensure to get exactly the anodizing results you want – even when using recycled aluminum.
How alloying elements affect anodized aluminum
One of the most important factors for surface finishing is how the aluminum is alloyed. This is because the alloying elements can affect the voltage response during anodizing. Therefore, better-than-best anodizing relies on a current-controlled anodizing process. Anodizers often apply the 312 rule (also known as the 720 rule) in order to determine the amount of current needed during the anodizing process. This is measured by the equation:
Rule of 312:
microns (of coating desired) × 3.12
Min to anodize = __________________________________________________________________
amps/sq dm
Rule of 720:
mils (of coating desired) × 720
Min to anodize = __________________________________________________________________
amps/sq ft
Based on the voltage response, aluminum alloys can be divided into three groups:
- Group 1 contains the alloys that are easy to anodize, including 1000 series almost pure aluminum, 5000 series aluminum-magnesium alloys, and easily extrudable 6000 series alloys, such as 6060 and 6063.
- Group 2 alloys tend to be more difficult and include e. g. 5000 and 6000 series alloys that have a high content of iron, copper, and chrome. This group also includes 3000 series and 7000 series alloys.
- Group 3 represents alloys that are very difficult to anodize and are specified for the 2000 series aluminum-copper alloys.
With every group, the amount of current needed (per square dm or square foot) in order to get 25 microns or 1 mil coating, grows. This is because, as the number of alloying elements in the microstructure increases, the resistance of the surface also increases—which means that the current needed to create a specific coating thickness will have to increase, too.
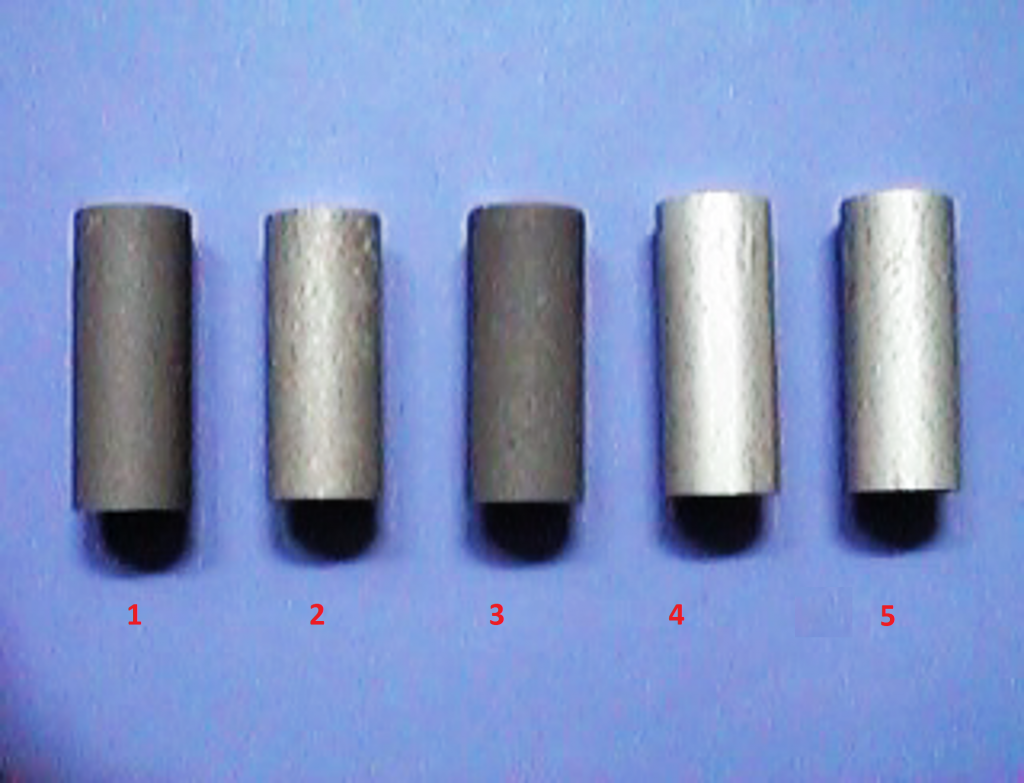
The image above shows five commonly used aluminum alloys that have been cast into rods and then hard anodized using the exact same process parameters for each. They were all anodized separately on a titanium rack, preventing any differences in their voltage variation from affecting one another during anodizing. Therefore, each of these rods should have the same final thickness layer after anodizing, but that is not the case. From left to right, the results are as follows:
- The aluminum-silicon alloy (7% silicon) sample has a layer thickness of 46 microns. The layer is dark and contains a lot of silicon embedded into the oxide film.
- The aluminum-copper alloy (4% copper) sample has a layer thickness of 27 microns. The layer is light grey and patchy.
- The aluminum-silicon alloy (5% silicon) sample has the same appearance as the first aluminum-silicon alloy (7% silicon) sample but had a smaller layer thickness of 39 microns.
- The aluminum alloy with 5% zinc has a layer thickness of 52 microns. The sample had a very bright and beautiful surface appearance.
- The final sample corresponds to 6063 and is an aluminum-silicon alloy (0.5% silicon and magnesium). It has a layer thickness of 52 microns and features a light grey appearance, which is typical for this type of aluminum.
Why does the alloy affect the anodizing result?
These results raise the question: why does the same amount of current not result in the same oxide layer thickness and appearance? Because the polarization of the surface is dependent on the surface constituents (alloying elements and their intermetallic phases) and how they behave during anodizing.
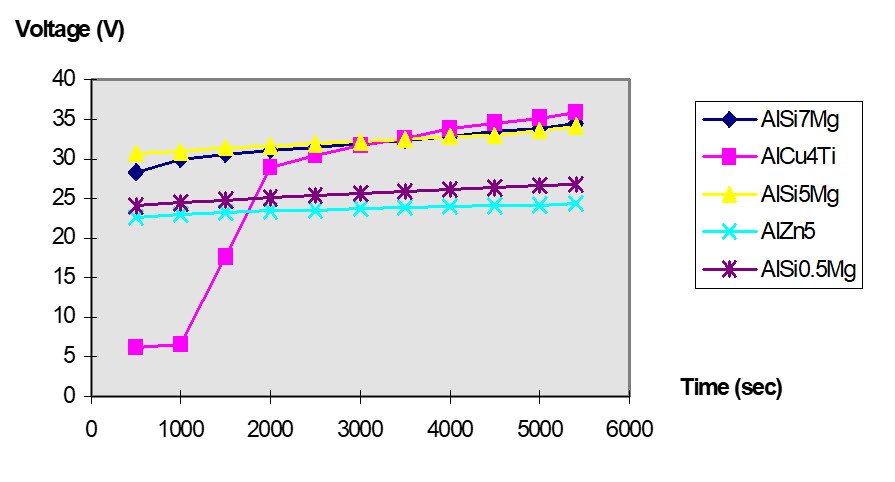
In the five aluminum alloy samples, the two alloys with the thickest layer (AlZn5 and Alsi0.5Mg) have the lowest voltage response and use the same current density. In other words, the current flows easily, the surface polarization is low, and because of this, a thick, uniform, and homogeneous layer is formed.
Comparing the two aluminum-silicon alloys (5% and 7% silicon), the silicon found in the microstructure occurs as pure silicon particles. The particles create small resistances, increasing the total resistance of the aluminum surface. In this way, the particles increase the surface activation potential, leading to a higher voltage response.
The aluminum-copper alloy behaves a little differently. Here, the copper atoms will migrate through the barrier layer faster than aluminum, because copper has a higher conductivity. At first, the current flows with almost no resistance, which can be seen in the very low voltage response at the beginning of the process. Then, as the process continues, the copper in the grain boundaries will start to dissolve. However, because these copper atoms are large, they start to have difficulties in getting to the surface and will then re-absorb in the oxide. Because of this, the resistance will start to increase, as seen by the increase in voltage over time.
How impurities affect anodized recycled aluminum
The samples previously discussed illustrate how the anodizing behavior changes with different alloys. Recycled aluminum also has the risk of higher impurities entering the alloy, which creates additional challenges, particularly when anodizing. Therefore, it is important to check the content of the main alloying elements (Si, Cu, Mg, Zn, Mn), the secondary elements (Fe, Cr, Ni, Pb, Sn, Ti, etc.), and the minor elements (Li, Sr, Sb, Ca, Bi, etc.).
For example, consider how iron impurities interfere with the final anodizing result. A common method to separate aluminum from other scrap materials is the eddy current separation method. Even after separation, very small amounts of ferrous metals and other contaminants can remain in the scrap when it finally reaches the melting stage. Even though the iron content is almost always <1% in these alloys, it can still cause difficulties in getting a uniform surface appearance.
When molten, iron is entirely soluble in aluminum. Thus, when the ingots are cooled, the microstructure will contain intermetallic compounds, such as iron-rich aluminum phases. A small percentage of iron mixed into the aluminum melt may not be of any particular concern for the microstructure, but can be an issue for the final application, as the aluminum-iron phase may reduce the ductility and machinability of the aluminum, as well as affect the appearance of the anodic layer on the surface. This means that if there is an increase in impurities during the recycling process, the behavior of an aluminum alloy cannot be predicted if its composition is not precisely known.
Let’s make anodizing more sustainable!
New EU regulations and supply chain laws force companies to make business more sustainable. And many people WANT to be part of the sustainable change! But sustainable change is also a question of knowledge and expertise. For many of us, it is an engineering problem: How can we produce the same products we have been selling for decades in a new, environmentally-friendly way?
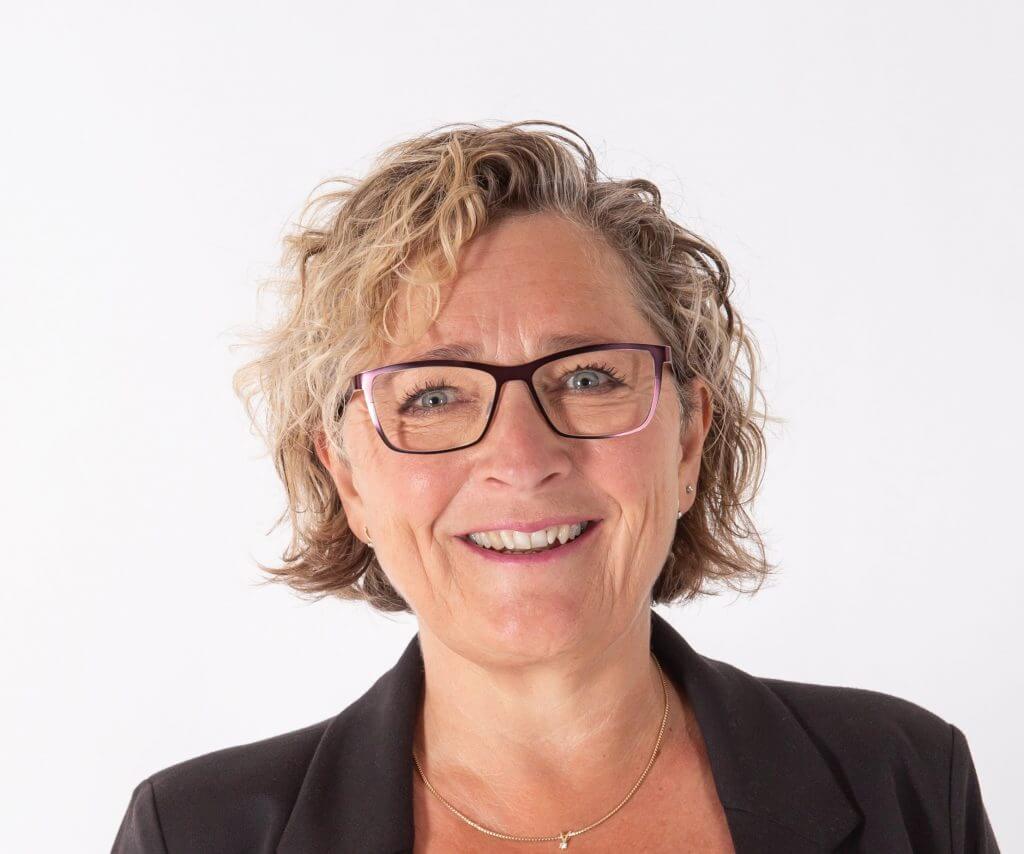
I want to answer this question with my 25+ years of experience in the anodizing industry! For this, I have created a live online course on how to create avoid the 5 most common mistakes when anodizing recycled aluminum. I hope to see you there!